The First Magnitude Stars
By Nigel Foster
This article is based on a Christmas quiz which I originally inflicted on members of Knowle Astronomical Society in December 2004. I chose the first magnitude stars as the theme for that quiz because I believed that the basic facts about these leading luminaries of the firmament (how big, how far away, how hot etc.) would be almost as well known as those (say) for the bodies in our own solar system. As I started to research the subject however, I first became disappointed at the obvious extent of my own ignorance and then surprised at the apparent extent of disagreement between the various sources I consulted. The fact of the matter is that the state of our knowledge about these, the most familiar of the stars, is evolving as rapidly as it is in many other areas of astronomy. For that reason I hope that the article will prove of even more interest than I first envisaged. If anything below is incorrect or becomes out of date (as I am sure it will over time) I would be most grateful if you would let me know. The rest of the article is presented in its original quiz format.
Question 1: Which is the brightest of the first magnitude stars?
Answer: Sirius at magnitude -1.46
An easy question to start with, although the answer does assume a definition of the term “first magnitude star” which requires some explanation. The magnitude scale was first invented by the Greek astronomer Hipparchus in the second century BC. He simply divided all of the stars in the sky into six categories depending on their apparent brightness. The brightest stars were of the first magnitude, the next brightest of the second magnitude and so on down to the faintest visible stars which were of the sixth magnitude in Hipparchus’ system. This was pretty much all there was to say on the subject for a very long time. The invention of the telescope at the start of the seventeenth century however, made it apparent that there were many millions of stars fainter than the sixth magnitude and by the middle of the nineteenth century, with the subject of astronomy advancing rapidly it was realised that there was a need for a more formal definition of stellar brightness that allowed for a continuous range of possible values, rather than just six discrete numbers. Early photometric measurements established that a first magnitude star is about 100 times as bright (based on measured incident light flux) as a sixth magnitude star and in 1856 Norman Pogson used this fact to adapt Hipparchus’ system to produce the magnitude scale that is still in use today. Pogson’s scale is logarithmic, with every interval of one magnitude equating to a variation in brightness by a factor of just over 2.5 times. Based on this continuous scale, any star with a magnitude of between 5.5 and 6.5 is now considered to be of the sixth magnitude, any star with a magnitude of between 4.5 and 5.5 is considered to be of the fifth magnitude and so on. Under this logic a first magnitude star ought to have a magnitude in the range 0.5 to 1.5. The problem is of course that there are nine stars with magnitude lower than 0.5 and the scale even has to be extended into negative territory to cater for the four brightest. However it would be rather pedantic of me to inform you that Betelgeuse and not Sirius is the brightest of the first magnitude stars. It is customary therefore to extend the definition of a first magnitude star to be any star with a magnitude lower than 0.5. There is one remaining caveat and that is (for a reason that should be obvious): to be considered first magnitude, a star must also be more than 93 million miles away!
Question 2: Which is the faintest of the first magnitude stars?
Answer: Regulus at magnitude 1.35
Regulus is the faintest of the 21 stars that satisfy the above definition. At one stage a 22nd star – Adhara in Canis Major was also thought to be of the first magnitude. The most recent measurements however have moved Adhara’s magnitude back from 1.49 to 1.50, so that it is relegated once more to head the list of second magnitude stars. The constellation of Canis Major therefore has the distinction of possessing both the brightest first and the brightest second magnitude star.
Question 3: Which is the closest first magnitude star?
Answer: Rigil Kentaurus (Alpha Centauri) at 4.4 light years (about 26 trillion miles)
The distance to Alpha Centauri was first determined by the Scottish astronomer Thomas Henderson in 1839. He used the only direct technique available for measuring distances in space, namely that of trigonometric parallax. Because of the vast distances of the stars, measurement of stellar parallax had eluded astronomers for many centuries until Friedrich Bessel’s famous breakthrough with 61 Cygni in 1838. Henderson’s measurements on Alpha Centauri were actually made earlier than those of Bessel but he did not manage to reduce his data until some months after Bessel had already gone to print.
Question 4: Which is the furthest first magnitude star?
Answer: Deneb at about 1,600 light years
Deneb is so far away that its parallax is too small to be obtained by methods currently at our disposal, so 1,600 light years is simply our current best estimate of its distance. Following the pioneering efforts of Bessel, Henderson and others such as Struve in the mid-19th century, Earth based astronomers over the next 150 years only managed to push the parallax method out to about 50 light years with any sort of accuracy. A huge leap forward came in 1989 with the launch of ESA’s “Hipparcos” satellite, whose name honours that great astronomer of antiquity and is an acronym of the somewhat contrived mission title: “High Precision Parallax Collecting Satellite. Hipparcos accurately measured the parallax of over a million stars, 120,000 of them with unprecedented, milliarcsecond accuracy and pushed the limit for the parallax method out to at least 500 light years.
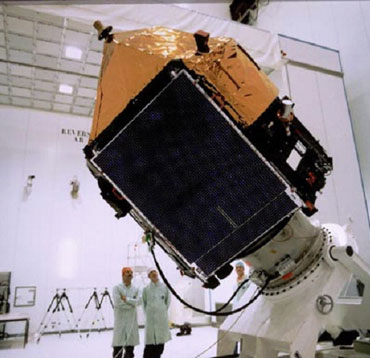 |
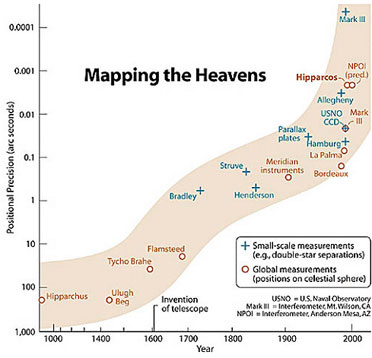 |
ESA images: Hipparcos Satellite and chart showing progress in measuring stellar parallax from the time of Hipparchus (about 150BC) to the time of Hipparcos.
Apart from Deneb only three other first magnitude stars were believed prior to Hipparcos to lie at distances greater than this and in each case the Hipparcos measurements led to a substantial change in the estimated distance. Rigel moved in from 900 to 733 light years, Betelgeuse moved in from 520 to 427 light years and Antares moved out from 520 to 604 light years.
Question 5: How many constellations have more than one first magnitude star?
Answer: Three (Orion, Crux and Centaurus)
Only three of the 88 constellations possess as many as two first magnitude stars. All the others have one or none. The three are Orion (with Rigel and Betelgeuse), Centaurus (with Rigil Kentaurus and Hadar) and Crux (with Acrux and Mimosa). As noted above, Canis Major comes close to being a fourth.
Question 6: Which pair of first magnitude stars are closest together?
Answer: Acrux and Mimosa (Alpha and Beta Crucis)
The two brightest stars of Crux (the Southern Cross) are just over four degrees apart, fractionally closer together than the next closest pair, Rigil Kentaurus and Hadar (Alpha and Beta Centauri), which themselves lie less than ten degrees away from the Crux pair in the southern sky.
Question 7: Are there more first magnitude stars north or south of the celestial equator?
Answer: There are 11 south of the celestial equator and 10 to the north
The southern skies are therefore marginally better endowed than the northern skies in terms of first magnitude stars. However the fact that the three brightest stars in the sky, Sirius, Canopus and Rigil Kentaurus all lie well south of the celestial equator (with the latter two never visible from the UK), leaves Northern Hemisphere observers feeling they have much the worst of the deal.
Question 8: Which are the most northerly and southerly first magnitude stars?
Answer: Capella (46o North) and Acrux (63o South)
The Southern Hemisphere also comes off best in terms of circumpolar stars (i.e. those that never set and therefore can be seen all night, every night). Whereas only Capella, Deneb and Vega are circumpolar from the Midlands of England, five stars are circumpolar from Sydney and Cape Town. These are the Crux and Centaurus pairs and Achernar in Eridanus. From much of New Zealand these five are comfortably above the horizon all of the time and also joined by Canopus.
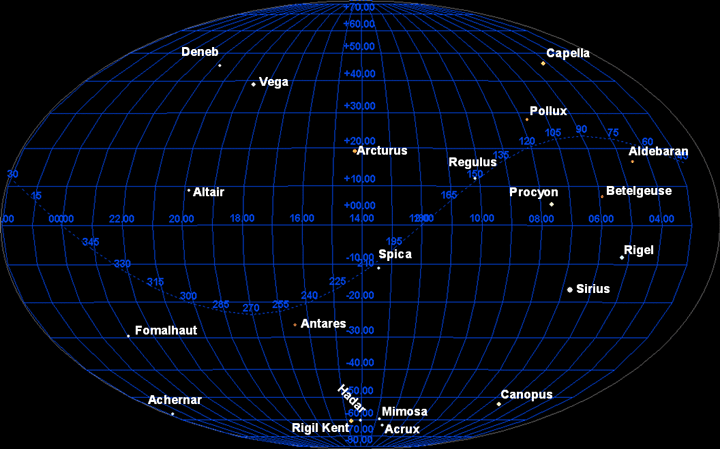
Image produced by Graystel software
Question 9: Which is the best season for seeing first magnitude stars?
Answer: Winter (with 8 stars)
The answer to this question is of course more subjective than some of the others, but it’s probably fair to say that a greater number of first magnitude stars are at their most prominent during Winter than at any other time of year. The eight normally regarded as winter stars are the six stars Sirius, Rigel, Aldebaran, Capella, Pollux and Procyon which form a rough hexagon surrounding a seventh, Betelgeuse, together with Canopus to the south.
Question 10: Which is the poorest season for seeing first magnitude stars?
Answer: Autumn (with just two: Fomalhaut and Achernar)
Again this is a matter of opinion but most observers would probably agree that autumn skies are the most barren in terms of bright stars. Dividing the remaining eleven first magnitude stars between Spring and Summer is likely to provoke more debate, since Arcturus and the two Centaurus stars can be considered borderline. However antipodean astronomers will probably consider the question rather academic since, as already noted, the latter two are for many of them circumpolar.
Question 11: How many of the first magnitude stars are variable?
Answer: Only Betelgeuse is markedly variable (ranging between magnitude +0.2 and +1.2), although another five are usually listed as variable and many of the others (probably the majority) are slight or suspected variables
For the record, the five other definite variables are: Aldebaran, Antares, Spica, Hadar and Mimosa.
Question 12: How many of the first magnitude stars are binary or multiple systems?
Answer: At least 13 – between 40 and 50 suns contribute to the light of the 21 first magnitude stars
The systems with the most members are Aldebaran, which is thought to have at least three dwarf companions and Capella which is a close binary orbited by a dwarf star which is itself double. The Acrux system also may have four components, although it is uncertain whether the fourth member is gravitationally bound to the others. Known triple stars in the list include Rigil Kentaurus, whose faintest member, the 11th magnitude red dwarf Proxima Centauri is the closest individual star of all, apart from our own Sun. Proxima Centauri is so distant from its two much brighter companions that it may only be a temporary member of that system. Famous doubles amongst the first magnitude stars include Sirius which has an eighth magnitude white dwarf companion. In the answers to the remaining questions I have ignored those members which do not contribute a significant percentage of the total light output of their system.
Question 13: Which first magnitude star has the shortest rotational period?
Answer: Altair rotates in 10 hours (compared with 25 days for the Sun)
Altair has a rotational velocity of 210 kilometres per second which is about 100 times that of the Sun. The extremely rapid rate at which the Altair is spinning about its axis causes it to bulge at the equator and Altair’s equatorial diameter is thought to be about 14% greater than its polar diameter. Even more extreme is Regulus, with a rotational velocity of about 350 kilometres per second and a bulge of 35%. The fact that Regulus is a larger star than Altair (about 3.5 times the Sun’s diameter compared with 1.5 times for Altair) means that it has a longer rotational period of about 16 hours. Even Regulus is not the most distorted star: Achernar, about 10 times as large as the Sun, rotates with a velocity slightly faster than that of Altair which causes it to distort by a massive 56%. Achernar is shedding significant amounts of material as a result of its rapid rotation, but it is calculated that it would need to be spinning twice as fast in order to disintegrate completely. At the other extreme Aldebaran is thought to have one of the lowest rotational velocities, possibly similar to that of the Sun meaning that with a diameter of over 40 times that of the Sun it may take as long as two years to rotate once about its axis, although there appears to be considerable uncertainty about this. The largest stars probably have rotational periods of many years, but despite this one of them, as we shall see later, is probably even more misshapen than Achernar.
Question 14: Which first magnitude star has the greatest proper motion?
Answer: Rigil Kentaurus (3.69 arcseconds per year)
As might logically be expected the closest star to us is the one that is moving fastest across our line of sight, although it still takes as much as 1,000 years to change its position by as much as one degree, relative to the other stars in the sky. The really interesting question is: which one is second fastest? Plotting the proper motions of the first magnitude stars against their distances in light years, one sees the expected rapid decrease until beyond 100 light years, values are typically below 0.1 arcseconds per year.
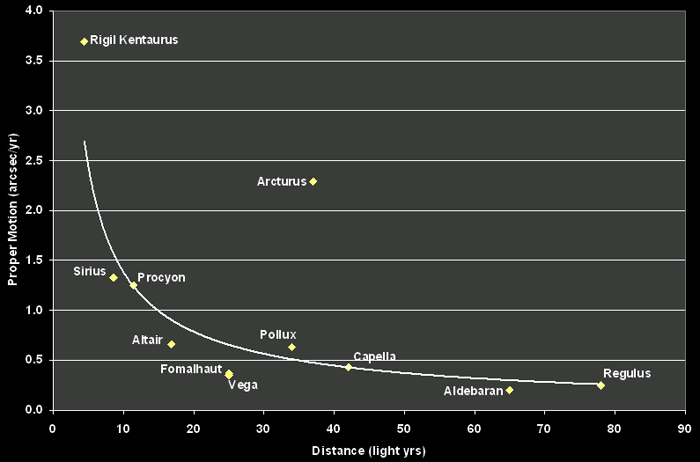
There is however one striking anomaly and that is Arcturus, which despite only being eighth closest has a much higher proper motion than any first magnitude star other than Alpha Centauri. Arcturus is believed to be a Population II or “Halo” star with an orbit highly inclined to the plane of the Milky Way, so that it is cutting across our path at a velocity of around 100 kilometres per second. Ordinary Population I stars like the Sun by comparison tend to meander along in the galactic plane moving in roughly the same direction as each other with relative velocities of a few tens of kilometres per second at most. The low metallicity of Arcturus supports this theory as Population II stars tend to be less contaminated than Population I stars with the heavy elements produced by the death of preceding stellar generations. Spectroscopic studies show that the iron content of Arcturus’ atmosphere is only 20% of that of the Sun.
Question 15: Which is the most massive first magnitude star?
Answer: The four stars of the Acrux system have a combined mass of about 40x that of our Sun, but the heaviest individual star is Deneb, weighing in at about 25 solar masses
Stars with ten solar masses or more are very rare and have a prodigious power output compared with our own Sun. The most distant of the first magnitude stars are bright because they are massive and powerful.
Super-heavyweights: stars with 10 or more solar masses:-
Debeb |
25 |
Betelgeuse |
12 |
Rigel |
17 |
Spica A |
11 |
Acrux A1 |
14 |
Hadar A |
11 |
Mimosa |
14 |
Acrux A2 |
10 |
Acrux B |
13 |
Canopus |
10 |
Antares |
12 |
Hadar B |
10 |
All of the above stars are more than 250 light years away
Question 16: Which is the least massive first magnitude star?
Answer: The lightest component of Rigil Kentaurus has a mass less than that of our own Sun and the other component is only slightly heavier than the Sun
The closest first magnitude stars are only bright because of their proximity to us and are generally rather modest in terms of mass and power output. Apart from the Rigil Kentaurus pair, only two other first magnitude stars, Altair and Procyon have masses less than twice that of the Sun. All four of these stars lie with 20 light years.
Lightweights: stars with < 2 solar masses:-
(excluding dwarf members)
|
Solar masses |
Distance (light years) |
Rigil Kentaurus B |
0.9 |
4 |
Rigil Kentaurus A |
1.1 |
4 |
Altair |
1.7 |
17 |
Procyon |
1.8 |
11 |
Question 17: Which is the biggest first magnitude star?
Answer:The revised distance estimates from the Hipparcos mission mean that Antares has now overtaken Betelgeuse which was previously thought to be the first magnitude star with the largest diameter
With the development of interferometry about a hundred years ago it became possible to measure the angular diameter of some stars. The first successful measurement took place in 1920 when the angular diameter of Betelgeuse was measured at 44 milliarcseconds.
Other direct methods of measuring angular diameters have also been successfully employed. For instance timings of lunar occultations of stars close to the ecliptic (e.g. Antares). Knowledge of a star’s angular diameter, together with its distance enables its actual diameter to be calculated. In many cases however angular diameters are too small to be measured and stellar diameters (as with masses) can only be estimated from theoretical considerations. Betelgeuse has comfortably the largest angular diameter of any star. As previously noted Betelgeuse is the only marked variable amongst the first magnitude stars and during the period of its light variations its diameter is estimated to pulsate between 480 and 760 times that of the Sun. There is only one other contender for the title of largest first magnitude star and that is Antares. The revised distance estimates for both stars resulting from the Hipparcos mission (see above) mean that Antares has in fact now overtaken Betelgeuse.
The Rest.... |
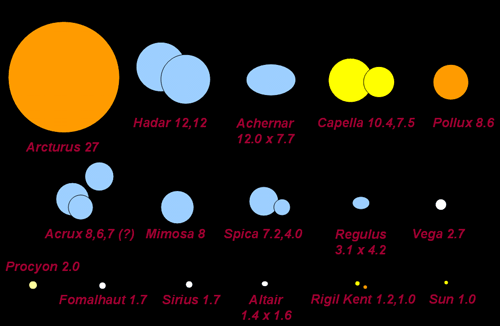 |
Antares is quite probably also the most misshapen with its equatorial diameter, 830 times that of the Sun, exceeding its polar diameter by 60%. Antares may not even be truly ellipsoidal. Lunar occultation studies indicate that it may in fact be egg shaped. Antares is so large that if it were to replace the Sun at the centre of our solar system its atmosphere would extend almost out to the orbit of Saturn. With a mass of only 12 times that of the Sun however Antares’ atmosphere is far more rarified than any vacuum we can create on Earth. After Antares and Betelgeuse the next largest stars are the other supergiants; Deneb, Rigel and Canopus, all with diameters more than 60 times that of the Sun. The sizes of the ‘ordinary’ giants range from 43 solar diameters in the case of Aldebaran down to 9 solar diameters in the case of Pollux. The largest and most powerful main sequence stars such as the two components of Hadar probably have diameters twelve times that of the Sun and lower down the main sequence Rigil Kentaurus B is estimated to have a diameter comparable to that of the Sun.
Question 18: Which is the hottest first magnitude star?
Answer: Both Mimosa and Acrux (A) are type B0 stars with temperatures of about 28,000 °K
From studies of their spectra, astronomers place the stars into different categories known as “spectral types”. The commonest spectral types are O, B, A, F, G, K & M. The principal feature behind this categorisation is the colour at which a star emits most of its radiation. A star’s colour is a direct indicator of its surface temperature and the hottest stars are the blue-white stars belonging to spectral type “O”. Amongst the first magnitude stars, there are none belonging to type O, but there are several belonging to the next hottest type, which is type “B”. Amongst these Mimosa and Acrux A are probably the hottest with surface temperatures around 28,000°K (compared with a mere 5,800°K for the Sun).
Question 19: Which is the coolest first magnitude star?
Answer: Betelgeuse.
Another close run thing between the two red supergiants in the group. Both belong to spectral type “M” but Betelgeuse is in a lightly later sub-class (i.e. M2 compared with M1 for Antares) and so with a surface temperature of 3,100 °K is probably just a shade cooler than Antares.
Question 20: Which is the most powerful first magnitude star?
Answer: Deneb
The first step in answering this question is to re-base visual magnitudes as if the stars were all at a common distance. The distance chosen by astronomers for this purpose is 32.6 light years or 10 parsecs (one parsec or 3.26 light years is the distance corresponding to a stellar parallax of one arcsecond).
Apparent Visual Magnitudes and Luminosities |
|
Absolute Visual Magnitudes and Luminosities |
|
Absolute Visual Magnitudes and Luminosities |
|
Adding in Infa-red..... |
|
And Ultra-violet.... |
|
The resulting re-based magnitudes are known as “absolute” magnitudes. Having performed this exercise we note that Sirius is now actually fainter than Regulus and only just first magnitude, and that Rigil Kentaurus is now an insignificant fourth magnitude star. On the other hand, Deneb now shines with the light of a thick crescent moon and five other stars are brighter than Venus. These five are Rigel, Canopus, Antares, Betelgeuse and Hadar.
Amongst these, Hadar consists of two more or less equal blue giants and as we have already seen many of the other stars are also multiple systems. Separating out the light contribution of all system members and placing them in their correct order we see that Rigel, which shines with the light of 40,000 Suns, is in clear second place behind Deneb which is 60,000 times as luminous. Canopus (15,000 times as luminous) heads the rest of the field. This is not the end of the story however. Our eyes are only tuned to receive a very limited part of the electromagnetic spectrum and to fully answer the question we need to consider power output across the entire range of wavelengths. The red supergiants, Antares and Betelgeuse emit copious amounts of infra-red and the hot blue stars of the upper main sequence emit most of their energy at ultra-violet wavelengths. Having accounted for these contributions, Deneb is still way in front 120,000 times as powerful as the Sun, but the blue giants of the Hadar system are neck and neck with Rigel for second place with about 50,000 times the power output of the Sun.
Astronomers attempt to make sense of the very wide range of power outputs and temperatures exhibited by stars by plotting the values of each these properties against each other on the same chart. This type of chart is called a Hertzsprung Russell (HR) diagram and it offers a great deal of insight about stellar evolution. The HR diagram for the first magnitude stars is shown below. As can be seen the majority of them lie within a band running from top right to bottom left. This band is known as the “main sequence” and main sequence stars are those which are still burning the initial supply of hydrogen fuel at their core. The Sun which is a very ordinary main sequence star is also shown on the diagram by way of comparison. The stars at the top left of the main sequence (e.g. Acrux, Mimosa, Hadar and Spica) are comparatively young (“young” in this context meaning millions rather than billions of years old). Massive stars such as these are known to consume their primordial hydrogen very rapidly and once this fuel is exhausted they start fusing helium and other heavier elements. At this stage they swell dramatically in size to become “supergiants” and at the same time their outer atmospheres cool significantly. As has already been seen, the first magnitude stars include several examples of such supergiants, including Antares and Betelgeuse.
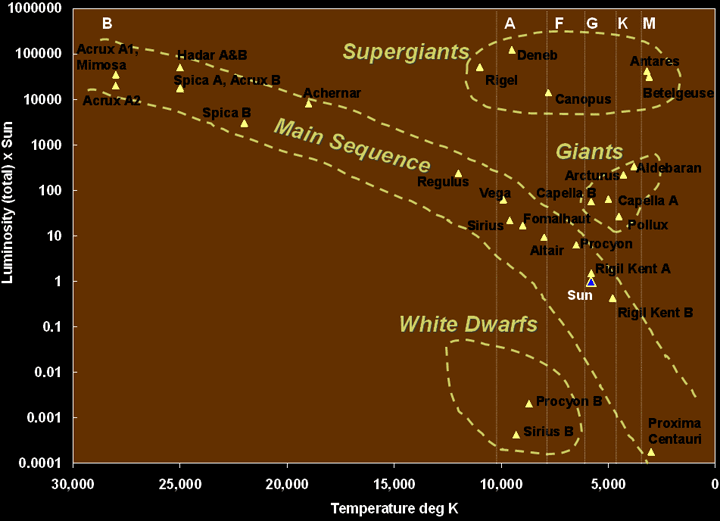
Further down the main sequence we encounter less massive stars such as Vega, Sirius and Fomalhaut, which are not vastly more luminous than the Sun and which will take a much longer period of time to exhaust their hydrogen. Eventually though they will end the main sequence phase of their lives and swell and cool to join the ranks of the ordinary giants half way down the right hand side of the diagram. First magnitude representatives amongst the ordinary giants include Arcturus and Aldebaran and our Sun will also become just such a star in about five billion years time. Finally, at the bottom right the diagram shows three of the most well known dwarf components of the first magnitude star systems - the main sequence red dwarf Proxima Centauri and the degenerate post-main sequence white dwarf companions of Sirius and Procyon.
Appendix 1. Postscript on Hadar (Beta Centauri)
To illustrate my initial comments about the rapidly evolving state of our knowledge about the first magnitude stars, there has been some recent news of an instance where it has been shown that even Hipparcos got the wrong result for the distance of one of the first magnitude stars.
Hadar: correction to Hipparcos distance
(Astronomy November 2004 article by Ken Croswell) |
|
The two components of Hadar revolve around each other in 357 days, which is of course very similar to the Earth’s orbital period of just over 365 days. As the diagram shows this fact seems to have confused Hipparcos into selecting a different component of the double star for the two parallax measurements from opposite sides of the Earth’s orbit. This resulted in the estimated parallax of Hadar being too small and the estimated distance too great at 525 light years. A team of Australian and European astronomers led by John Davis of Sydney University realised this problem and in 2004 used spectroscopy and interferometry to estimate the correct distance of Hadar at 330 light years. Follow up work in 2006 led to a revision of this estimate to 352 light years and all of the parameters of the Hadar system used in the preparation of this article are taken or derived from this latest work.
Appendix 2: Some Great Websites
In addition to sources named above, the following are gratefully acknowledged with sincere apologies for any omissions.
Stars, created by Jim Kaler, Professor Emeritus of Astronomy at the University of Illinois is an authoritative and comprehensive source on hundreds of the brightest stars and much else. It is also being constantly expanded (at the rate of one star per week) and updated. “Stars” is a truly superb resource for anyone interested in astronomy.
SolStation.com is another absolute mine of information – particularly on the closest stars to us.
The Internet Stellar Database by Roger Wilcox contains an extremely comprehensive list of data for each star (again particularly the closest); all recalibrated for the Hipparcos parallax measurements.
Appendix 3: Data Table
|